|
Research
This page is getting old. I will try to find time to update this page soon...
Formation and Evolution of Galaxies
How do galaxies form and evolve? --- this is one of the central questions of
extragalactic astronomy today. I am particularly interested in the formation of
massive galaxies. A good fraction of massive galaxies are quiescent
with little signs of on-going star formation even at high redshifts
and they are known to be physically compact.
How do such objects form? In order to address the question, I am working on
near-IR spectra of massive quiescent galaxies taken with MOIRCS/Subaru and MOSFIRE/Keck,
trying to constrain their formation scenarios. Also, to gain deeper insights into
the formation of massive compact galaxies, I measure sizes of z~4
massive galaxies with IRCS/Subaru assisted by laser-guided adaptive optics system.
Suprisingly, these galaxies are even smaller than their counterparts at z~2, which
actually deepens the question. In addition, deep near-IR spectroscopy of the most
distant massive quiescent galaxies reveal that their dynamical masses. The previous
redshift record was z=2.7, so this is a giant leap in redshift! Surprisingly,
the core mass of these galaxies are similar to that of massive galaxies today,
which further deepens the question of the massive galaxy formation. Work is in progress
to observe more quiescent galaxies at higher redshifts.
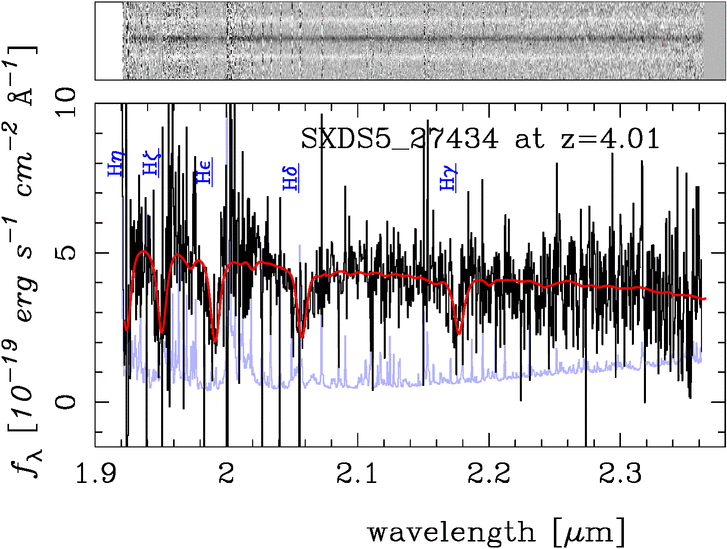 |
Near-IR spectrum of the most distant massive quiescent galaxy at z=4.01
(12 billion years ago) taken with Keck/MOSFIRE. Note the prominent absorption
lines due to Hydrogen (Tanaka et al. 2019, ApJL).
|
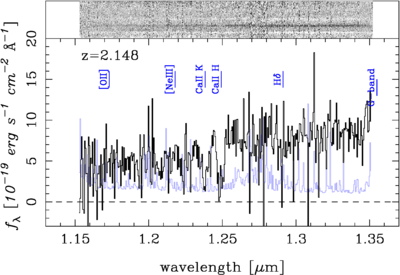 |
MOSFIRE spectrum of a massive quiescent galaxy in a proto-cluster at z=2.1
(Tanaka et al. in prep).
|
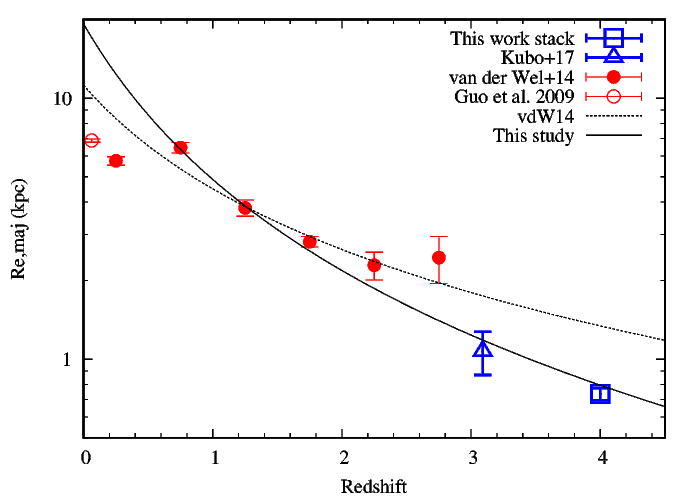 |
Size evolution of massive quiescent galaxies. The data point at z=4 is from IRCS/SUbaru
and it shows that these quiescent galaxies at z=4 are extremely compact
(Kubo, Tanaka et al. in prep).
|
Near-Field Cosmology
The lambda-dominated cold dark matter model, a model in which the dark energy is
the most dominant energy in the universe, is now widely accepted as a standard
cosmological model. It has been extensively tested on a large-scale, but
there are a few possible flaws on a small-scale. One of them is the missing
satellite problem; the number of dwarf galaxies in the Local Group is much smaller
than that predicted by LCDM simulations. The problem here is not just
the discrepancy in the number, but the fact that the problem has been tested
only in the Local Group. Is the Local Group halo a typical halo in the Universe?
Who knows? Any cosmological problems have to be addressed statistically!
We have started a project to observe nearby galaxies with HSC to address
the problem with an unprecedented statistical accuracy. Our pilot
observation was very successful (Tanaka et al, ApJ 2018) and we are now extending the sample.
In addition to the missing satellite problem, our data (as well as data from
HSC SSP) can also be used to detect diffuse stellar tidal streams, which are
a clear signature of dynamical interactions between galaxies and are a good
probe of galaxy-scale assembly. This project has evolved to a citizen science
project and we will launch a website to get the public people involved in this project.
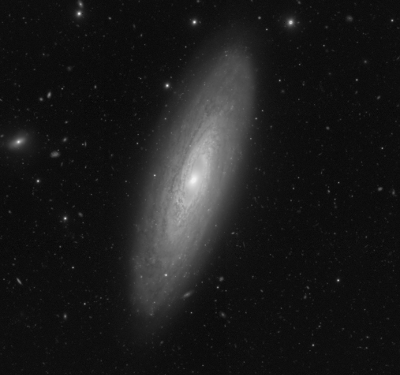 |
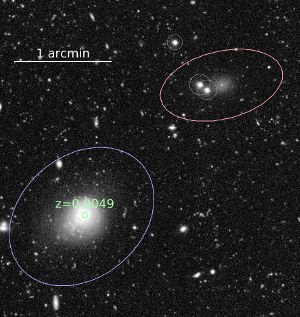 |
Left: NGC779 (d~22Mpc)
Right: identified diffuse dwarf satellite galaxies
|
Citizen Science
In addition to the missing satellite problem, HSC-SSP data are extremely
useful for identifying diffuse tidal features around galaxies.
These are the remnants of galaxy-galaxy interactions and are thus a useful
ptobe of how galaxies evovle over the cosmic time. The current framework
of galaxy evolution suggests that the mergers are one of the main modes of
the galaxy growth.
However, it is hard to find interacting galaxies because there are so many galaxies
out there. A way around it is to ask citizen scientists to help us.
We launched the first citizen science project in astronomy in Japan, GALAXY CRUISE,
and it has been going extremely well so far. Even NHK (national TV station)
filmed us!
Active Galactic Nuclei
One of the long-standing issues in numerical simulations of galaxy formation
is the over-cooling problem; in the center of massive halos, cooling is
more efficient than heating and galaxies covert an enormous amount of gas into
stars and become way too massive (much more massive than real galaxies).
Super-massive black holes now likely exist in most of the massive galaxies
and they are suspected to heat the gas by releasing energy of the infalling material
and prevent the over-cooling.
However, detailed physics of this process is largely unknown. Whether this AGN feedback
is really important for galaxy evolution or not can be tested by correlating, e.g.,
AGN activity and physical properties of the host galaxies.
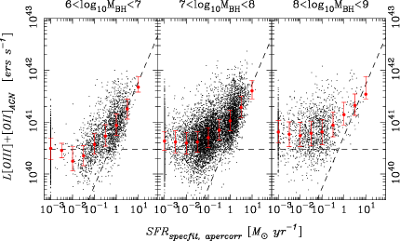 |
AGN power vs star formation rate of the host galaxies in the local Universe (Tanaka 2012, PASJ, 64, 37).
The dashed lines are selection functions and we miss objects on the right/below the lines.
|
Photometric Redshifts
Spectroscopy is a standard technique to measure distances of objects at
cosmological distances, but it is a very expensive observation.
Photometric redshift is a much cheaper technique to infer redshifts of galaxies
using photometric information such as magnitudes and colors. It has become an essential
tool for on-going/upcoming large imaging surveys. There are broadly two classes
of photo-z techniques;
template fitting and machine-learning. I am interested in both, but so far
I have primarily worked on the template fitting technique with a novel idea to
achieve good accuracy. I apply a set of Bayesian priors on physical properties
of galaxies, which effectively evolves the templates with redshifts in
an observationally motivated way. The code also estimates physical properties
of galaxies such as star formation rate and stellar mass in a fully consistent
manner. The code is extensively used in the on-going HSC survey.
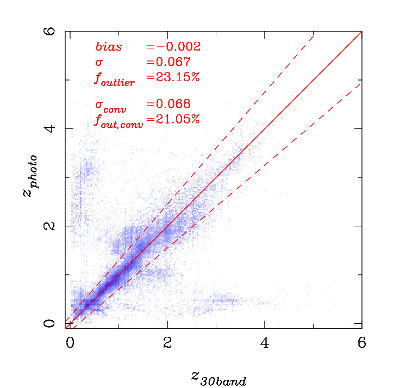 |
HSC 5-band photo-z vs 30-band photo-z from COSMOS down to i=25. No clipping is applied.
The accuracy is still not as great as I would like and I am trying to include
information like sizes and morphologies in the photo-z estimates.
(Tanaka 2015, ApJ, 801, 20, Tanaka et al. 2018, PASJ, 70, 9)
|
Data Processing Pipeline
Both HSC and PFS generate a huge amount of data. For reference, the data volume of one of
the recent internal data release from HSC is about 400TB! It is essential to build a data processing
pipeline to efficiently process the data. As part of my duty work, I am involved in the pipeline
development of HSC and PFS. My expertize is the instrument chacterization/calibration
(Doi, Tanaka, et al. AJ, 2010, 139, 1628) using stars, but I also develop algorithms that are useful
for my own science.
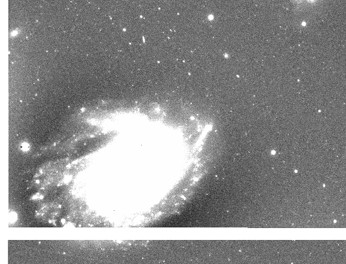 |
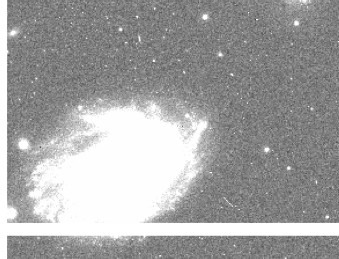 |
Left: original sky subraction for HSC.
Right: revised algorithm.
|
|
|